The electronic structure of phosphotungstic (H₃PW₁₂O₄₀) heteropolyacids modified by Fe²⁺ cation
DOI:
https://doi.org/10.5604/01.3001.0014.7530Keywords:
heteropolyacids, density functional theory, electronic structure, catalysis, NOCV-SRAbstract
In this paper the influence of substituting the tungsten atom with an iron ion in the primary structure of the phosphotungstic heteropolyacid with the Keggin anion structure was investigated. Characterization of the electronic structure of the modified heteropolyacid was performed using: population analysis according to NBO scheme, total (TDOS) and partial (PDOS) density of states spectra, energy and chemical character of frontier orbitals (HOMO / LUMO) and the size of the HOMO-LUMO band gap. Additionally, the mechanism of interaction between the Fe²⁺ with H₂O molecule, acting as a chemical reaction medium, was investigated. Most cases showed a significant effect of the introduced transition metal ion (Fe²⁺) on the above-mentioned properties in relation to the nonmodified heteropolyacid H₃PW₁₂O₄₀.
Downloads
References
Wei Y, Xu B, Barnes CL, Peng Z. An efficient and convenient reaction protocol to organoimido derivatives of polyoxometalates. Journal of the American Chemical Society. 2001;123(17):4083–4084. doi: https://doi.org/10.1021/ja004033q. Google Scholar
Vazylyev M, Sloboda-Rozner D, Haimov A, Maayan G, Neumann R. Strategies for oxidation catalyzed polyoxometalates at the interference of homogeneous and heterogeneous catalysis. Topics in Catalysis. 2005;34:93–99. doi: https://doi.org/10.1007/s11244-005-3793-5. Google Scholar
Shigeta S, Mori S, Kodama E, Kodama J, Takahashi K, Yamase T. Broad spectrum anti-RNA virus activities of titanium and vanadium substituted polyoxotungstates. Antiviral Research. 2003;58(3):265–271. doi: https://doi.org/10.1016/S0166-3542(03)00009-3. Google Scholar
Hierle R, Badan J, Zyss J. Growth and characterization of a new material for nonlinear optics: methyl- 3-nitro-4-pyridine-1-oxide (POM). Journal of Crystal Growth. 1984;69(2-3):545–554. doi: https://doi.org/10.1016/0022-0248(84)90366-X. Google Scholar
Qiu W, Zheng Y, Haralampides KA. Study on a novel POM-based magnetic photocatalyst: Photocatalytic degradation and magnetic separation. Chemical Engineering Journal. 2007;125(3):165–176 doi: https://doi.org/10.1016/j.cej.2006.08.025. Google Scholar
Mizuno N, Misono M. Heterogeneous catalysis. Chemical Reviews. 1998;98(1):199–218. doi: https://doi.org/10.1021/cr960401q. Google Scholar
Kozhevnikov IV. Catalysis by heteropoly acids and multicomponent poly oxometalates in liquid-phase reactions. Chemical Reviews. 1998;98(1):171–198. doi: https://doi.org/10.1021/cr960400y. Google Scholar
Okuhara T, Mizuno N, Misono M. Catalytic chemistry of heteropoly compounds. Advances in Catalysis. 1996;41:113–252. doi: https://doi.org/10.1016/S0360-0564(08)60041-3. Google Scholar
Kozhevnikov IV. Heteropoly acids and related compounds as catalysts for fine chemical synthesis. Catalysis Reviews: Science and Engineering. 1995;37(2):311–352. doi: https://doi.org/10.1080/01614949508007097. Google Scholar
Casarini D, Centi G, Jiru P, Lena V, Tvaruzkova Z. Reactivity of molybdovanadophosphoric acids: influence of the presence of vanadium in the primary and secondary structure. Journal of Catalysis. 1993;143(2):325–344. doi: https://doi.org/10.1006/jcat.1993.1280. Google Scholar
Harrup MK, Hill CL. Polyoxometalate catalysis of the aerobic oxidation of hydrogen sulfide to sulfur. Inorganic Chemistry. 1994;33(24):5448–5455. doi: https://doi.org/10.1021/ic00102a017. Google Scholar
Neumann R, Abu-Gnim C. Alkene oxidation catalyzed by a ruthenium-substituted heteropolyanion, SiRu(L)W11O39: the mechanism of the periodate-mediated oxidative cleavage. Journal of the American Chemical Society.1990;112(16):6025–6031. doi: https://doi.org/10.1021/ja00172a018. Google Scholar
Weber RS. Molecular orbital study of C-H bond breaking during the oxidative dehydrogenation of methanol catalyzed by metal oxide surfaces. Journal of Physical Chemistry. 1994;98(11):2999–3005. doi: https://doi.org/10.1021/j100062a042. Google Scholar
Zhang FQ, Zhang XM, Wu HS, Jiao H. Structural and electronic properties of hetero-transition-metal Keggin anions: a DFT study of α/β-[XW12O40]n− (X = CrVI, VV, TiIV, FeIII, CoIII, NiIII, CoII, and ZnII) relative stability. Journal of Physical Chemistry A. 2007;111(1):111, 159–166. doi: https://doi.org/10.1021/jp064732a. Google Scholar
Maestre JM, Lopez X, Bo C, Poblet J-M, Casañ-Pastor N. Electronic and magnetic properties of α-Keggin anions: a DFT study of [XM12O40]n−, (M = W, Mo; X = AlIII, SiIV, PV, FeIII, CoII, CoIII) and [SiM11VO40]m− (M = Mo and W). Journal of the American Chemical Society. 2001;123(16):3749–3758. doi: https://doi.org/10.1021/ja003563j. Google Scholar
Zonnevijlle F, Tourné CM, Tourné GF. Preparation and characterization of heteropolytungstates containing group 3a elements. Inorganic Chemistry. 1982;21(7):2742–2750. doi: https://doi.org/10.1021/ic00137a041. Google Scholar
Nakajima K, Eda K, Himeno S. Effect of the central oxoanion size on the voltammetric properties of Keggin-type [XW12O40]n− (n = 2 − 6) complexes. Inorganic Chemistry. 2010;49(11):5212–5216. doi: https://doi.org/10.1021/ic1003353. Google Scholar
Altenau JJ, Pope MT, Prados RA, So H. Models for heteropoly blues: Degrees of valence trapping in vanadium(IV)- and molybdenum(V)-substituted Keggin anions. Inorganic Chemistry. 1975;14(2):417–421. doi: https://doi.org/10.1021/ic50144a042. Google Scholar
Keita B, Nadjo L. New oxometalate-based materials for catalysis and electrocatalysis. Materials Chemistry and Physics. 1989;22(1–2):77–103. doi: https://doi.org/10.1016/0254-0584(89)90032-1. Google Scholar
Sun W, Liu H, Kong J, Xie G, Deng J. Redox electrochemistry of Keggin type iridium-substituted heteropolytungstates and their electrocatalytic activity toward the reduction of nitrite ion. Journal of Electroanalytical Chemistry. 1997;437(1–2):67–76. doi: https://doi.org/10.1016/S0022-0728(97)00356-2. Google Scholar
Rong C, Anson FC. Simplified preparations and electrochemical behavior of two chromium-substituted heteropolytungstate anions. Inorganic Chemistry. 1994;33(6):1064–1070. doi: https://doi.org/10.1021/ic00084a016. Google Scholar
Maeda K, Katano H, Osakai T, Himeno S, Saito A. Charge dependence of one-electron redox potentials of Keggin-type heteropolyoxometalate anions. Journal of Electroanalytical Chemistry. 1995;389(1–2):167–173. doi: https://doi.org/10.1016/0022-0728(95)03872-E. Google Scholar
Dong S, Xi X, Tian M. Study of the electrocatalytic reduction of nitrite with silicotungstic heteropolyanion. Journal of Electroanalytical Chemistry. 1995;385(2):227–233. doi: https://doi.org/10.1016/0022-0728(94)03770-4. Google Scholar
Turbomole V6.3 2011 adoUoKa. Forschungszentrum Karlsruhe GmbH – Turbomole GmbH saf. http://www.turbomole.com. Google Scholar
Perdew JP, Burke K, Ernzerhof M. Generalized gradient approximation made simple. Physical Review Letters. 1996;77(18):3865–3868. doi: https://doi.org/10.1103/PhysRevLett.77.3865. Google Scholar
Slater JC. The self-consistent field for molecular and solids. Quantum theory of molecular and solids. Vol. 4. New York: McGraw-Hill; 1974. Google Scholar
Perdew JP, Wang Y. Pair-distribution function and its coupling-constant average for the spin-polarized electron gas. Physical Review B. Condensed Matter. 1992;46(20):12947–12954. doi: https://doi.org/10.1103/physrevb.46.12947. Google Scholar
Schaefer A, Horn H, Ahlrichs R. Pair-distribution function and its coupling-constant average for the spin-polarized electron gas. The Journal of Chemical Physics. 1992;97: 2571. Google Scholar
Schäfer A, Horn H, Ahlrichs R. Fully optimized contracted Gaussian basis sets for atoms Li to Kr. The Journal of Chemical Physics. 1992;97(4):2571–2577. doi: https://doi.org/10.1063/1.463096. Google Scholar
Pearson RG. Hard and soft acids and bases. Journal of the American Chemical Society. 1963;85(22):3533–3539. doi: https://doi.org/10.1021/ja00905a001. Google Scholar
Pearson RG. Hard and soft acids and bases, HSAB. Part 1: Fundamental principles. Journal of Chemical Education. 1968;45(9):581–586. doi: https://doi.org/10.1021/ed045p581. Google Scholar
Pearson RG. Hard and soft acids and bases, HSAB. Part II: Underlying theories. Journal of Chemical Education. 1968;45(10):643–648. doi: https://doi.org/10.1021/ed045p643. Google Scholar
Keggin JF. The structure and formula of 12-phosphotungstic acid. Proceedings of the Royal Society A. Mathematical, Physical and Engineering Sciences. 1934;144(851):75–100. doi: https://doi.org/10.1098/rspa.1934.0035. Google Scholar
Geneste G, Morillo J, Finocchi F. Adsorption and diffusion of Mg, O, and O2 on the MgO(001) flat surface. The Journal of Chemical Physics. 2005;122(17):174707. doi: https://doi.org/10.1063/1.1886734. Google Scholar
Idriss H, Barteau MA, Active sites on oxides: from single crystals to catalysts. Advances in Catalysis. 2000;45:261–331. doi: https://doi.org/10.1016/S0360-0564(02)45016-X. Google Scholar
Freund H-J, Pacchioni G. Oxide ultra-thin films on metals: new materials for the design of supported metal catalysts. Chemical Society Reviews. 2008;37(10):2224–2242. doi: https://doi.org/10.1039/B718768H. Google Scholar
Pacchioni G. Electronic interactions and charge transfers of metal atoms and clusters on oxide surfaces. Physical Chemistry Chemical Physics. 2013;15(6):1737–1757. doi: https://doi.org/10.1039/C2CP43731G. Google Scholar
Natorbs (version 3.0) – universal tool for computing natural (spin)orbitals and natural orbitals for chemical valence http://www.chemia.uj.edu.pl/~mradon/natorbs. Google Scholar
Nalewajski RF, Mrozek J, Formosinho SJ, Varandas AJC. Quantum mechanical valence study of a bond-breaking–bond-forming process in triatomic systems. International Journal of Quantum Chemistry. 1994;52(5):1153–1176. doi: https://doi.org/10.1002/qua.560520504. Google Scholar
Nalewajski RF, Mrozek J. Modified valence indices from the two-particle density matrix. International Journal of Quantum Chemistry. 1994;51(4):187-200. doi: https://doi.org/10.1002/qua.560510403. Google Scholar
Nalewajski RF, Mrozek J, Mazur G. Quantum chemical valence indices from the one-determinantal difference approach. Canadian Journal of Chemistry. 1996;74(6):1121–1130. doi: https://doi.org/10.1139/v96-126. Google Scholar
Nalewajski RF, Mrozek J, Michalak A. Two-electron valence indices from the Kohn-Sham orbitals. International Journal of Quantum Chemistry. 1997;61(3):589–601. doi: https://doi.org/10.1002/(SICI)1097-461X(1997)61:3<589::AID-QUA28>3.0.CO;2-2. Google Scholar
Nalewajski RF, Mrozek J, Michalak A. Exploring bonding patterns of molecular systems using quantum mechanical bond multiplicities. Polish Journal of Chemistry. 1998;72(2S):1779–1791. Google Scholar
Mitoraj M, Michalak A. Natural orbitals for chemical valence as descriptors of chemical bonding in transition metal complexes. Journal of Molecular Modeling. 2007;13(2):347–355. doi: https://doi.org/10.1007/s00894-006-0149-4. Google Scholar
Reed AE, Weinstock RB, Weinhold F. Natural population analysis. The Journal of Chemical Physics. 1985;83(2):735. doi: https://doi.org/10.1063/1.449486. Google Scholar
Mayer I. Charge, bond order and valence in the AB initio SCF theory. Chemical Physics Letters. 1983;97(3):270–274. doi: https://doi.org/10.1016/0009-614(83)80005-0. Google Scholar
Bridgeman AJ, Cavigliasso G, Ireland LR, Rothery J. The Mayer bond order as a tool in inorganic chemistry. Journal of the Chemical Society, Dalton Transactions. 2001;14: 2095–2108. doi: https://doi.org/10.1039/B102094N. Google Scholar
Fowe EP, Therrien B, Süss-Fink G, Daul C. Electron-structure calculations and bond order analysis using density functional theory of cationic dinuclear arene ruthenium complexes. Inorganic Chemistry. 2008;47(1):42–48. doi: https://doi.org/10.1021/ic7007914. Google Scholar
Klamt A, Schüürmann G. COSMO: a new approach to dielectric screening in solvents with explicit expressions for the screening energy and its gradient. Journal of Chemical Society, Perkin Transactions 2. 1993;5:799–805. doi: https://doi.org/10.1039/P29930000799. Google Scholar
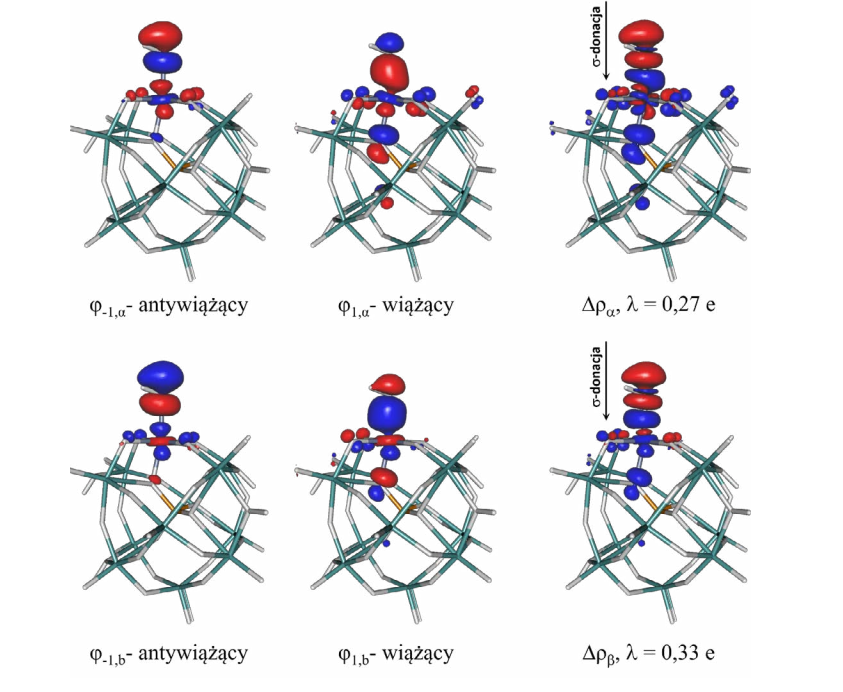
Downloads
Published
How to Cite
Issue
Section
License
Copyright (c) 2020 University of Applied Sciences in Tarnow, Poland & Author

This work is licensed under a Creative Commons Attribution-NonCommercial 4.0 International License.