Wykorzystanie symulacji Monte Carlo do oceny potencjału zastosowania nanowęgli gyroidalnych jako adsorbentów do wychwytu ditlenku siarki
DOI:
https://doi.org/10.55225/sti.619Słowa kluczowe:
ditlenek siarki, SO₂, adsorpcja, nanoporowate węgle gyroidalne, symulacje molekularneAbstrakt
Ditlenek siarki jest zanieczyszczeniem gazowym mogącym wywierać negatywny wpływ na środowisko naturalne oraz ludzkie zdrowie. Adsorpcja jest jedną z metod, którą można wykorzystać do jego usuwania. W ramach niniejszego badania wykorzystano symulacje Monte Carlo do przewidzenia właściwości adsorpcyjnych nanoporowatych węgli gyroidalnych względem SO₂. Układy tego typu stanowią nową obiecującą klasę szeroko rozumianych nanomateriałów węglowych. Wykorzystując serię węglowych struktur modelowych o geometrii gyroidalnej, w sposób systematyczny zbadano ilościowo wpływ różnic ich struktury porowatej na wychwyt ditlenku siarki. Dokonano również porównania z adsorpcją w nieuporządkowanych porach węgli aktywnych. Potwierdzono, że kluczowym parametrem jest rozmiar porów. Nanowęgle gyroidalne o węższych porach adsorbują większą ilość SO₂ przy jego niskiej prężności, a jednocześnie cała objętość ich porów może zostać zapełniona przy niższym ciśnieniu. Zwiększenie rozmiaru porów sprawia jednak, że rośnie maksymalna liczba moli ditlenku siarki pochłaniana przez jednostkę masy adsorbentu, ale jej osiągnięcie następuje przy wyższej prężności tego gazu. Z praktycznego punktu widzenia znaczenie ma przede wszystkim adsorpcja przy jego niskich ciśnieniach. W takich warunkach nanowęgle gyroidalne, zwłaszcza te o wąskich porach, jak GNC-04, mogą być efektywnymi adsorbentami SO₂.
Statystyka pobrań
Bibliografia
Hung Y-T, Ashner I. Sulfur dioxide emission and mitigation. In: Hung Y-T, Wang LK, Shammas NK, editors. Handbook of Environment and Waste Management. Vol. 3: Acid Rain and Greenhouse Gas Pollution Control. London: World Scientific Publishing; 2020. p. 627–658. https://doi.org/10.1142/9789811207136_0018. DOI: https://doi.org/10.1142/9789811207136_0018 Google Scholar
Kang H, Zhu B, van der A RJ, Zhu C, de Leeuw G, Hou X, Gao J. Natural and anthropogenic contributions to long-term variations of SO₂, NO₂, CO, and AOD over East China. Atmospheric Research. 2019;215:284–293. https://doi.org/10.1016/j.atmosres.2018.09.012. DOI: https://doi.org/10.1016/j.atmosres.2018.09.012 Google Scholar
Smith SJ, van Aardenne J, Klimont Z, Andres RJ, Volke A, Delgado Arias S. Anthropogenic sulfur dioxide emissions: 1850–2005. Atmospheric Chemistry and Physics. 2011;11:1101–1116. https://doi.org/10.5194/acp-11-1101-2011. DOI: https://doi.org/10.5194/acp-11-1101-2011 Google Scholar
Ren Y, Shen G, Shen H, Zhong Q, Xu H, Meng W, Zhang W, Yu X, Yun X, Luo Z, Chen Y, Li B, Cheng H, Zhu D, Tao S. Contributions of biomass burning to global and regional SO₂ emissions. Atmospheric Research. 2021;260:105709. https://doi.org/10.1016/j.atmosres.2021.105709. DOI: https://doi.org/10.1016/j.atmosres.2021.105709 Google Scholar
Jion MMF, Jannat JN, Mia Y, Ali A, Islam S, Ibrahim SM, Pal SC, Islam A, Sarker A, Malafaia G, Bilal M, Islam ART. A critical review and prospect of NO₂ and SO₂ pollution over Asia: Hotspots, trends, and sources. Science of the Total Environment. 2023;876:162851. https://doi.org/10.1016/j.scitotenv.2023.162851. DOI: https://doi.org/10.1016/j.scitotenv.2023.162851 Google Scholar
Fatima F, Fatima N, Amjad T, Anjum A, Afzal T, Riaz J, Razzaq H. A review on acid rain: An environmental threat. Pure and Applied Biology. 2021;10:301–310. https://doi.org/10.19045/bspab.2021.100032. DOI: https://doi.org/10.19045/bspab.2021.100032 Google Scholar
Twagirayezu G, Nizeyimana JC, Irumva O, Ntakiyimana C, Uwimpaye F, Nyirandayisabye R, In: Manzi HP, Hakuzweyezu T. A critical review of acid rain: Causes, effects, and mitigation measures. In: Walag AMP, editor. Novel Perspectives of Geography, Environment and Earth Sciences Vol. 6. BP International; 2023. p. 23–40. https://doi.org/10.9734/bpi/npgees/v6/5127A. DOI: https://doi.org/10.9734/bpi/npgees/v6/5127A Google Scholar
Abbasi T, Poornima P, Kannadasan T, Abbasi SA. Acid rain: Past, present, and future. International Journal of Environmental Engineering. 2013;5:229–272. https://doi.org/10.1504/IJEE.2013.054703. DOI: https://doi.org/10.1504/IJEE.2013.054703 Google Scholar
Grennfelt P, Engleryd A, Forsius M, Hov Ø, Rodhe H, Cowling E. Acid rain and air pollution: 50 years of progress in environmental science and policy. Ambio. 2020;49:849–864. https://doi.org/10.1007/s13280-019-01244-4. DOI: https://doi.org/10.1007/s13280-019-01244-4 Google Scholar
Liu Z, Yang J, Zhang J, Xiang H, Wei H. A bibliometric analysis of research on acid rain. Sustainability. 2019;11:3077. https://doi.org/10.3390/su11113077. DOI: https://doi.org/10.3390/su11113077 Google Scholar
Orellano P, Reynoso J, Quaranta N. Short-term exposure to sulphur dioxide (SO₂) and all-cause and respiratory mortality: A systematic review and meta-analysis. Environment International. 2021;150:106434. https://doi.org/10.1016/j.envint.2021.106434. DOI: https://doi.org/10.1016/j.envint.2021.106434 Google Scholar
Khajeamiri Y, Sharifi S, Moradpour N, Khajeamiri A. A review on the effect of air pollution and exposure to PM, NO₂, O₃, SO₂, CO and heavy metals on viral respiratory infections. Journal of Air Pollution and Health. 2020;5:243–258. https://doi.org/10.18502/japh.v5i4.6445. DOI: https://doi.org/10.18502/japh.v5i4.6445 Google Scholar
Khalaf EM, Mohammadi MJ, Sulistiyani S, Ramírez-Coronel AA, Kiani F, Jalil AT, Almulla AF, Asban P, Farhadi M, Derikondi M. Effects of sulfur dioxide inhalation on human health: A review. Reviews on Environmental Health. 2022;22:331–337. https://doi.org/10.1515/reveh-2022-0237. DOI: https://doi.org/10.1515/reveh-2022-0237 Google Scholar
Chen Z, Liu N, Tang H, Gao X, Zhang Y, Kan H, Deng F, Zhao B, Zeng X, Sun Y, Qian H, Liu W, Mo J, Zheng X, Huang C, Sun C, Zhao Z. Health effects of exposure to sulfur dioxide, nitrogen dioxide, ozone, and carbon monoxide between 1980 and 2019: A systematic review and meta-analysis. Indoor Air. 2022;32:e13170. https://doi.org/10.1111/ina.13170. DOI: https://doi.org/10.1111/ina.13170 Google Scholar
Huang J, Zheng W, Huang H, Ran Y, Liu Y, Huang P. Particulate matter, nitrogen dioxide, and sulfur dioxide and their associations with allergic skin diseases: A systematic review and meta-analysis. Atmospheric Pollution Research. 2023;14:101804. https://doi.org/10.1016/j.apr.2023.101804. DOI: https://doi.org/10.1016/j.apr.2023.101804 Google Scholar
Fazakas E, Neamtiu IA, Gurzau ES. Health effects of air pollutant mixtures (volatile organic compounds, particulate matter, sulfur and nitrogen oxides) – a review of the literature. Reviews on Environmental Health. 2023;39:459–478. https://doi.org/10.1515/reveh-2022-0252. DOI: https://doi.org/10.1515/reveh-2022-0252 Google Scholar
Liu W, Cai M, Long Z, Tong X, Li Y, Wang L, Zhou M, Wei J, Lin H, Yin P. Association between ambient sulfur dioxide pollution and asthma mortality: Evidence from a nationwide analysis in China. Ecotoxicology and Environmental Safety. 2023;249:114442. https://doi.org/10.1016/j.ecoenv.2022.114442. DOI: https://doi.org/10.1016/j.ecoenv.2022.114442 Google Scholar
Chen R, Zhang T, Guo Y, Wang J, Wei J, Yu Q. Recent advances in simultaneous removal of SO₂ and NOx from exhaust gases: Removal process, mechanism and kinetics. Chemical Engineering Journal. 2021;420:127588. https://doi.org/10.1016/j.cej.2020.127588. DOI: https://doi.org/10.1016/j.cej.2020.127588 Google Scholar
Pasichnyk M, Stanovsky P, Polezhaev P, Zach B, Šyc M, Bobák M, Jansen JC, Přibyl M, Bara JE, Friess K, Havlica J, Gin DL, Noble RD, Izák P. Membrane technology for challenging separations: Removal of CO₂, SO₂ and NOx from flue and waste gases. Separation and Purification Technology. 2023;323:124436. https://doi.org/10.1016/j.seppur.2023.124436. DOI: https://doi.org/10.1016/j.seppur.2023.124436 Google Scholar
Kumar L, Jana SK. Advances in absorbents and techniques used in wet and dry FGD: A critical review. Reviews in Chemical Engineering. 2022;38:843–880. https://doi.org/10.1515/revce-2020-0029. DOI: https://doi.org/10.1515/revce-2020-0029 Google Scholar
Chandran K, Kait CF, Wilfred CD, Zaid HFM. A review on deep eutectic solvents: Physiochemical properties and its application as an absorbent for sulfur dioxide. Journal of Molecular Liquids. 2021;338:117021. https://doi.org/10.1016/j.molliq.2021.117021. DOI: https://doi.org/10.1016/j.molliq.2021.117021 Google Scholar
Hanif MA, Ibrahim N, Jalil AA. Sulfur dioxide removal: An overview of regenerative flue gas desulfurization and factors affecting desulfurization capacity and sorbent regeneration. Environmental Science and Pollution Research. 2020;27:27515–27540. https://doi.org/10.1007/s11356-020-09191-4. DOI: https://doi.org/10.1007/s11356-020-09191-4 Google Scholar
Zhao M, Xue P, Liu J, Liao J, Guo J. A review of removing SO₂ and NOX by wet scrubbing. Sustainable Energy Technologies and Assessments. 2021;47:101451. https://doi.org/10.1016/j.seta.2021.101451. DOI: https://doi.org/10.1016/j.seta.2021.101451 Google Scholar
Islam A, Teo SH, Ng CH, Taufiq-Yap YH, Choong SYT, Awual R. Progress in recent sustainable materials for greenhouse gas (NOx and SOx) emission mitigation. Progress in Materials Science. 2023;132:101033. https://doi.org/10.1016/j.pmatsci.2022.101033. DOI: https://doi.org/10.1016/j.pmatsci.2022.101033 Google Scholar
Yu H, Shan C, Li J, Hou X, Yang L. Alkaline absorbents for SO₂ and SO₃ removal: A comprehensive review. Journal of Environmental Management. 2024;366:121532. https://doi.org/10.1016/j.jenvman.2024.121532. DOI: https://doi.org/10.1016/j.jenvman.2024.121532 Google Scholar
Silas K, Ghani WAWAK, Choong TSY, Rashid U. Carbonaceous materials modified catalysts for simultaneous SO₂/NOx removal from flue gas: A review. Catalysis Reviews. 2018;61:134–161. https://doi.org/10.1080/01614940.2018.1482641. DOI: https://doi.org/10.1080/01614940.2018.1482641 Google Scholar
Sepehrian M, Anbia M, Hedayatzadeh MH, Yazdi F. SO₂ dry-based catalytic removal from flue gas leading to elemental sulfur production: A comprehensive review. Process Safety and Environmental Protection. 2024;182:456–480. https://doi.org/10.1016/j.psep.2023.11.077. DOI: https://doi.org/10.1016/j.psep.2023.11.077 Google Scholar
Hou Y, Chen Y, He X, Wang F, Cai Q, Shen B. Insights into the adsorption of CO₂, SO₂ and NOx in flue gas by carbon materials: A critical review. Chemical Engineering Journal. 2024;490:151424. https://doi.org/10.1016/j.cej.2024.151424. DOI: https://doi.org/10.1016/j.cej.2024.151424 Google Scholar
Wang H, Yuan B, Hao R, Zhao Y, Wang X. A critical review on the method of simultaneous removal of multi-air-pollutant in flue gas. Chemical Engineering Journal. 2019;378:122155. https://doi.org/10.1016/j.cej.2019.122155. DOI: https://doi.org/10.1016/j.cej.2019.122155 Google Scholar
Kong D, Lian L, Wang Y, Hussain A, Liu Y. A review on carbon-based and coordination polymer-based materials for adsorption of SO₂ from flue gas. Chemical Engineering Journal. 2024;500:157089. https://doi.org/10.1016/j.cej.2024.157089. DOI: https://doi.org/10.1016/j.cej.2024.157089 Google Scholar
Xie J, Wang D, Liu L, Shao T, Zhou H, Zhang D. An overview of flue gas SO₂ capture technology based on absorbent evaluation and process intensification. Industrial & Engineering Chemistry Research. 2024;63:6066–6086. https://doi.org/10.1021/acs.iecr.4c00405. DOI: https://doi.org/10.1021/acs.iecr.4c00405 Google Scholar
Zewdie DT, Bizualem YD, Nurie AG. A review on removal CO₂, SO₂, and H₂S from flue gases using zeolite based adsorbents. Discover Applied Sciences. 2024;6:331. https://doi.org/10.1007/s42452-024-05989-w. DOI: https://doi.org/10.1007/s42452-024-05989-w Google Scholar
Wen W, Wen C, Wang D, Zhu G, Yu J, Ling P, Xu M, Liu T. A review on activated coke for removing flue gas pollutants (SO₂, NOx, Hg0, and VOCs): Preparation, activation, modification, and engineering applications. Journal of Environmental Chemical Engineering. 2024;12:111964. https://doi.org/10.1016/j.jece.2024.111964. DOI: https://doi.org/10.1016/j.jece.2024.111964 Google Scholar
Bamdad H, Hawboldt K, MacQuarrie S. A review on common adsorbents for acid gases removal: Focus on biochar. Renewable and Sustainable Energy Reviews. 2018;81:1705–1720. https://doi.org/10.1016/j.rser.2017.05.261. DOI: https://doi.org/10.1016/j.rser.2017.05.261 Google Scholar
Aimikhe VJ , Eyankware OE. Adsorbents for noxious gas sequestration: State of the art. Journal of Scientific Research & Reports. 2019;25:1–21. https://doi.org/10.9734/JSRR/2019/v25i1-230176. DOI: https://doi.org/10.9734/jsrr/2019/v25i1-230176 Google Scholar
Ge JC, Yoon SK, Choi NJ. Application of fly ash as an adsorbent for removal of air and water pollutants. Applied Sciences. 2018;8:1116. https://doi.org/10.3390/app8071116. DOI: https://doi.org/10.3390/app8071116 Google Scholar
Hasan HF, Al-Sudani FT, Albayati TM, Salih IK, Hharah HN, Majdi HS, Saady NMC, Zendehboudi S, Amari A, Gheni SA. Solid adsorbent material: A review on trends of post-combustion CO2 capture. Process Safety and Environmental Protection. 2024;182:975–988. https://doi.org/10.1016/j.psep.2023.12.025. DOI: https://doi.org/10.1016/j.psep.2023.12.025 Google Scholar
Gupta NK, López-Olvera A, González-Zamora E, Martínez-Ahumada E, Ibarra IA. Sulfur dioxide capture in metal-organic frameworks, metal-organic cages, and porous organic cages. ChemPlusChem. 2022;87:e202200006. https://doi.org/10.1002/cplu.202200006. DOI: https://doi.org/10.1002/cplu.202200006 Google Scholar
Zafari R, Mendonça FG, Baker RT, Fauteux-Lefebvre C. Efficient SO₂ capture using an amine-functionalized, nanocrystalline cellulose-based adsorbent. Separation and Purification Technology. 2023;308:122917. https://doi.org/10.1016/j.seppur.2022.122917. DOI: https://doi.org/10.1016/j.seppur.2022.122917 Google Scholar
Nie Y, Dai J, Hou Y, Zhu Y, Wang C, He D, Mei Y. An efficient and environmentally friendly process for the reduction of SO₂ by using waste phosphate mine tailings as adsorbent. Journal of Hazardous Materials. 2020;388:121748. https://doi.org/10.1016/j.jhazmat.2019.121748. DOI: https://doi.org/10.1016/j.jhazmat.2019.121748 Google Scholar
Yang Y, Hao X, Sun Z, Chen Y, Xu Z, Zhao W. Fabrication of metal oxides-based adsorbents for SO₂ capture with equimolar adsorption. Chemical Engineering Journal. 2024;486:150106. https://doi.org/10.1016/j.cej.2024.150106. DOI: https://doi.org/10.1016/j.cej.2024.150106 Google Scholar
Guerrero-Sánchez J, Munoz-Pizza DM, Takeuchi N. Silicene as an efficient way to fully inactivate the SO₂ pollutant. Applied Surface Science. 2019;479:847–851. https://doi.org/10.1016/j.apsusc.2019.02.078. DOI: https://doi.org/10.1016/j.apsusc.2019.02.078 Google Scholar
Tong S, Zhu J, Wang Z, Yan J. Highly selective SO₂ capture by triazine-functionalized triphenylamine-based nanoporous organic polymers. ACS Applied Materials & Interfaces. 2024;16:42717–42725. https://doi.org/10.1021/acsami.4c08905. DOI: https://doi.org/10.1021/acsami.4c08905 Google Scholar
Yu L, He M, Yao J, Xia Q, Yang S, Li J, Wang H. A robust aluminum-octacarboxylate framework with scu topology for selective capture of sulfur dioxide. Chemical Science. 2024;15:8530–8535. https://doi.org/10.1039/D4SC01877J. DOI: https://doi.org/10.1039/D4SC01877J Google Scholar
Ma Y, Li A, Wang C. Experimental study on adsorption removal of SO₂ in flue gas by defective UiO-66. Chemical Engineering Journal. 2023;455:140687. https://doi.org/10.1016/j.cej.2022.140687. DOI: https://doi.org/10.1016/j.cej.2022.140687 Google Scholar
Awad AM, Jalab R, Benamor A, Nasser MS, Ba-Abbad MM, El-Naas M, Mohammad AW. Adsorption of organic pollutants by nanomaterial-based adsorbents: An overview. Journal of Molecular Liquids. 2020;301:112335. https://doi.org/10.1016/j.molliq.2019.112335. DOI: https://doi.org/10.1016/j.molliq.2019.112335 Google Scholar
Jamshed A, Iqbal A, Ali S, Ali S, Mamoon M. A quick review on the applications of nanomaterials as adsorbents. MOJ Ecology & Environmental Sciences. 2023;8:86–89. https://doi.org/10.15406/mojes.2023.08.00278. DOI: https://doi.org/10.15406/mojes.2023.08.00278 Google Scholar
Qin H, Liu Y, Liu H, Di S, Zhu S. Application and research progress of nanomaterials as adsorbents in environment field. In: Tharini J, Thomas S, editors. Carbon Nanomaterials and their Composites as Adsorbents. Cham: Springer; 2024. p. 105–134. https://doi.org/10.1007/978-3-031-48719-4_7. DOI: https://doi.org/10.1007/978-3-031-48719-4_7 Google Scholar
Liu X, Li Y, Chen Z, Yang H, Cai Y, Wang S, Chen J, Hu B, Huang Q, Shen C, Wang X. Advanced porous nanomaterials as superior adsorbents for environmental pollutants removal from aqueous solutions. Critical Reviews in Environmental Science and Technology. 2023;53:1289–1309. https://doi.org/10.1080/10643389.2023.2168473. DOI: https://doi.org/10.1080/10643389.2023.2168473 Google Scholar
Balakumar S, Mahesh N, Kamaraj M, Saranya T, Babu PS, Aravind J, Kim W, Govarthanan M. Customized carbon composite nanomaterials for the mitigation of emerging contaminants: A review of recent trends. Carbon Letters. 2024;34:1091–1114. https://doi.org/10.1007/s42823-024-00715-3. DOI: https://doi.org/10.1007/s42823-024-00715-3 Google Scholar
Bansal RC, Goyal M. Adsorpcja na węglu aktywnym. Warszawa: Wydawnictwo Naukowe PWN; 2009. Google Scholar
Alengebawy A, Deka TJ, Ban S, Chen Z, Osman AI, Yap P-S, Ai P. Carbonaceous sorbents for hydrogen purification. In: Rahimpour MR, Makarem MA, Kiani P, editors. Hydrogen Purification and Separation. Boca Raton: CRC Press; 2024. https://doi.org/10.1201/9781003382522-15. DOI: https://doi.org/10.1201/9781003382522-15 Google Scholar
Serafin J, Dziejarski B. Activated carbons – preparation, characterization and their application in CO2 capture: A review. Environmental Science and Pollution Research. 2024;31:40008–40062. https://doi.org/10.1007/s11356-023-28023-9. DOI: https://doi.org/10.1007/s11356-023-28023-9 Google Scholar
Sharma G, Sharma S, Kumar A, Lai CW, Naushad M, Iqbal SJ, Stadler FJ. Activated carbon as superadsorbent and sustainable material for diverse applications. Adsorption Science & Technology. 2022;2022:1. https://doi.org/10.1155/2022/4184809. DOI: https://doi.org/10.1155/2022/4184809 Google Scholar
Reza S, Yun CS, Afroze S, Radenahmad N, Bakar MSA, Saidur R, Taweekun J, Azad AK. Preparation of activated carbon from biomass and its’ applications in water and gas purification, a review. Arab Journal of Basic and Applied Sciences. 2020;27:208–238. https://doi.org/10.1080/25765299.2020.1766799. DOI: https://doi.org/10.1080/25765299.2020.1766799 Google Scholar
Shi L, Zhu Z, Wu N, Chang Y, Yue L, An L. Adsorption characteristics of SO₂ onto novel activated carbon fixed bed: Kinetics, isotherms, thermodynamics and washing regeneration. Environmental Technology. 2023;45:5182–5203. https://doi.org/10.1080/09593330.2023.2283810. DOI: https://doi.org/10.1080/09593330.2023.2283810 Google Scholar
Abdulrasheed AA, Jalil AA, Triwahyono S, Zaini MAA, Gambo Y, Ibrahim M. Surface modification of activated carbon for adsorption of SO₂ and NOX: A review of existing and emerging technologies. Renewable and Sustainable Energy Reviews. 2018;94:1067–1085. https://doi.org/10.1016/j.rser.2018.07.011. DOI: https://doi.org/10.1016/j.rser.2018.07.011 Google Scholar
Sheng H, Zhao X, Wang S, He T, Zhang J, He S, Huang Y. Nitrogen-doped high-surface-area activated carbon: Innovative adsorbent for enhanced SO₂ and benzene removal. Journal of Industrial and Engineering Chemistry. 2024;144:463–475. https://doi.org/10.1016/j.jiec.2024.09.041. DOI: https://doi.org/10.1016/j.jiec.2024.09.041 Google Scholar
Wang T, Tian S, Li G, Zhang L, Sheng M, Ren W. Molecular simulation of gas adsorption in shale nanopores: A critical review. Renewable and Sustainable Energy Reviews. 2021;149:111391. https://doi.org/10.1016/j.rser.2021.111391. DOI: https://doi.org/10.1016/j.rser.2021.111391 Google Scholar
Datar A, Witman M, Lin L-C. Monte Carlo simulations for water adsorption in porous materials: Best practices and new insights. AIChE Journal. 2021;67:e17447. https://doi.org/10.1002/aic.17447. DOI: https://doi.org/10.1002/aic.17447 Google Scholar
Salahshoori I, Wang Q, Nobre MAL, Mohammadi AH, Dawi EA, Khonakdar HA. Molecular simulation-based insights into dye pollutant adsorption: A perspective review. Advances in Colloid and Interface Science. 2024;333:103281. https://doi.org/10.1016/j.cis.2024.103281. DOI: https://doi.org/10.1016/j.cis.2024.103281 Google Scholar
Obliger A, Bousige C, Coasne B, Leyssale J-M. Development of atomistic kerogen models and their applications for gas adsorption and diffusion: A mini-review. Energy & Fuels. 2023;37:1678–1698. https://doi.org/10.1021/acs.energyfuels.2c03633. DOI: https://doi.org/10.1021/acs.energyfuels.2c03633 Google Scholar
Li J, Wang Y, Chen Z, Rahman SS. Simulation of adsorption-desorption behavior in coal seam gas reservoirs at the molecular level: A comprehensive review. Energy & Fuels. 2020;34:2619–2642. https://doi.org/10.1021/acs.energyfuels.9b02815. DOI: https://doi.org/10.1021/acs.energyfuels.9b02815 Google Scholar
Tylianakis E, Froudakis GE. Grand canonical Monte Carlo method for gas adsorption and separation. Journal of Computational and Theoretical Nanoscience. 2009;6:335–348. https://doi.org/10.1166/jctn.2009.1040. DOI: https://doi.org/10.1166/jctn.2009.1040 Google Scholar
Steele W. Computer simulations of physical adsorption: A historical review. Applied Surface Science. 2002;196:3–12. https://doi.org/10.1016/S0169-4332(02)00038-7. DOI: https://doi.org/10.1016/S0169-4332(02)00038-7 Google Scholar
Binder K. Applications of Monte Carlo methods to statistical physics. Reports on Progress in Physics. 1997;60:487–559. https://doi.org/10.1088/0034-4885/60/5/001. DOI: https://doi.org/10.1088/0034-4885/60/5/001 Google Scholar
Furmaniak S, Terzyk AP, Gauden PA, Kowalczyk P, Szymański GS. Influence of activated carbon surface oxygen functionalities on SO₂ physisorption – Simulation and experiment. Chemical Physics Letters. 2013;578:85–91. https://doi.org/10.1016/j.cplett.2013.05.060. DOI: https://doi.org/10.1016/j.cplett.2013.05.060 Google Scholar
Peng X, Jain SK, Singh JK. Adsorption and separation of N2/CH₄/CO2/SO₂ gases in disordered carbons obtained using hybrid reverse Monte Carlo simulations. Journal of Physical Chemistry C. 2017;121:13457–13473. https://doi.org/10.1021/acs.jpcc.7b01925. DOI: https://doi.org/10.1021/acs.jpcc.7b01925 Google Scholar
Zhao R, Liu G, Wei G, Gao J, Lu H. Analysis of SO₂ physisorption by edge-functionalized nanoporous carbons using grand canonical Monte Carlo methods and density functional theory: implications for SO₂ removal. ACS Omega. 2021;6:33735–33746. https://doi.org/10.1021/acsomega.1c05000. DOI: https://doi.org/10.1021/acsomega.1c05000 Google Scholar
Maurya M, Singh JK. A grand canonical Monte Carlo study of SO₂ capture using functionalized bilayer graphene nanoribbons. Journal of Chemical Physics. 2017;146:044704. https://doi.org/10.1063/1.4974309. DOI: https://doi.org/10.1063/1.4974309 Google Scholar
Rahimi M, Babu DJ, Singh JK, Yang Y-B, Schneider JJ, Müller-Plathe F. Double-walled carbon nanotube array for CO2 and SO₂ adsorption. Journal of Chemical Physics. 2015;143:124701. https://doi.org/10.1063/1.4929609. DOI: https://doi.org/10.1063/1.4929609 Google Scholar
Yang Y-B, Rahimi M, Singh JK, Böhm MC, Müller-Plathe F. Adsorption and condensation of SO₂ in double-walled carbon nanotube arrays studied by Monte Carlo simulations and simple analytical models. Journal of Physical Chemistry C. 2016;120:7510–7521. https://doi.org/10.1021/acs.jpcc.5b08910. DOI: https://doi.org/10.1021/acs.jpcc.5b08910 Google Scholar
Nickmand Z, Aghamiri SF, Khozanie MRT, Sabzyan H. A Monte Carlo simulation of the adsorption of CO2 and SO₂ gases in pure and functionalized single walled carbon nanotubes. Separation Science and Technology. 2014;49:499–505. https://doi.org/10.1080/01496395.2013.862277. DOI: https://doi.org/10.1080/01496395.2013.862277 Google Scholar
Rahimi M, Singh JK, Müller-Plathe F. Adsorption and separation of binary and ternary mixtures of SO₂, CO2 and N2 by ordered carbon nanotube arrays: Grand-canonical Monte Carlo simulations. Physical Chemistry Chemical Physics. 2016;18:4112–4120. https://doi.org/10.1039/c5cp06377a. DOI: https://doi.org/10.1039/C5CP06377A Google Scholar
Yang Y-B, Hao Q, Müller-Plathe F, Böhm MC. Monte Carlo Simulations of SO₂, H2S, and CO2 adsorption in charged single-walled carbon nanotube arrays. Journal of Physical Chemistry C. 2020;124:5838–5852. https://doi.org/10.1021/acs.jpcc.9b10424. DOI: https://doi.org/10.1021/acs.jpcc.9b10424 Google Scholar
Chen S-Y, Hui Y, Yang Y-B. Monte Carlo simulations of adsorption and separation of binary mixtures of CO2, SO₂, and H2S by charged single-walled carbon nanotubes. Soft Materials. 2020;18:262–273. https://doi.org/10.1080/1539445X.2020.1729806. DOI: https://doi.org/10.1080/1539445X.2020.1729806 Google Scholar
Sun W, Lin L-C, Peng X, Smit B. Computational screening of porous metal-organic frameworks and zeolites for the removal of SO₂ and NOx from flue gases. AIChE Journal. 2014;60:2314–2323. https://doi.org/10.1002/aic.14467. DOI: https://doi.org/10.1002/aic.14467 Google Scholar
Vellamarthodika S, Gautam S. SO₂ adsorption in ZSM-22: Role of orientational disorder in the adsorbent. AIP Conference Procedings. 2024;2995:020028. https://doi.org/10.1063/5.0178213. DOI: https://doi.org/10.1063/5.0178213 Google Scholar
Chen E, Jia L, Jia X, Wei Q, Zhang L. Understanding the adsorption and separation of sulfur dioxide in flue gas by zeolitic imidazolate frameworks via molecular simulation. Chemical Physics Letters. 2021;778:138788. https://doi.org/10.1016/j.cplett.2021.138788. DOI: https://doi.org/10.1016/j.cplett.2021.138788 Google Scholar
Vellamarthodika S, Gautam S. Role of orientational disorder in ZSM-22 in the adsorption of SO₂. Molecular Physics. 2022;120:e2117663. https://doi.org/10.1080/00268976.2022.2117663. DOI: https://doi.org/10.1080/00268976.2022.2117663 Google Scholar
Song X-D, Wang S, Hao C, Qiu J-S. Investigation of SO₂ gas adsorption in metal-organic frameworks by molecular simulation. Inorganic Chemistry Communications. 2014;46:277–281. https://doi.org/10.1016/j.inoche.2014.06.003. DOI: https://doi.org/10.1016/j.inoche.2014.06.003 Google Scholar
Livas CG, Raptis D, Tylianakis E, Froudakis GE. Multiscale theoretical study of sulfur dioxide (SO₂) adsorption in metal-organic frameworks. Molecules. 2023;28:3122. https://doi.org/10.3390/molecules28073122. DOI: https://doi.org/10.3390/molecules28073122 Google Scholar
Wang H, Bai JQ, Yin Y, Wang SF. Experimental and numerical study of SO₂ removal from a CO2/SO₂ gas mixture in a Cu-BTC metal organic framework. Journal of Molecular Graphics and Modelling. 2020;96:107533. https://doi.org/10.1016/j.jmgm.2020.107533. DOI: https://doi.org/10.1016/j.jmgm.2020.107533 Google Scholar
Grubišić S, Dahmani R, Djordjević I, Sentić M, Hochlaf M. Selective adsorption of sulphur dioxide and hydrogen sulphide by metal-organic frameworks. Physical Chemistry Chemical Physics. 2023;25:954–965. https://doi.org/10.1039/d2cp04295a. DOI: https://doi.org/10.1039/D2CP04295A Google Scholar
Devaraj M, Pai SDKR, Badawi M, Pillai RS. Molecular simulation prediction on SO₂ gas adsorption in bipyridine ligand-based square-pillared MOFs. ACS Applied Nano Materials. 2024;7:16630–16638. https://doi.org/10.1021/acsanm.4c02680. DOI: https://doi.org/10.1021/acsanm.4c02680 Google Scholar
Schoen AH. Reflections concerning triply-periodic minimal surfaces. Interface Focus. 2012;2:658–668. https://doi.org/10.1098/rsfs.2012.0023. DOI: https://doi.org/10.1098/rsfs.2012.0023 Google Scholar
Werner JG, Hoheisel TN, Wiesner U. Synthesis and characterization of gyroidal mesoporous carbons and carbon monoliths with tunable ultralarge pore size. ACS Nano. 2014;8:731–743. https://doi.org/10.1021/nn405392t. DOI: https://doi.org/10.1021/nn405392t Google Scholar
Werner JG, Johnson SS, Vijay V, Wiesner U. Carbon-sulfur composites from cylindrical and gyroidal mesoporous carbons with tunable properties in lithium-sulfur batteries. Chemistry of Materials. 2015;27:3349–3357. https://doi.org/10.1021/acs.chemmater.5b00500. DOI: https://doi.org/10.1021/acs.chemmater.5b00500 Google Scholar
Werner JG, Scherer MRJ, Steiner U, Wiesner U. Gyroidal mesoporous multifunctional nanocomposites via atomic layer deposition. Nanoscale. 2014;6:8736–8742. https://doi.org/10.1039/c4nr01948b. DOI: https://doi.org/10.1039/C4NR01948B Google Scholar
Werner JG, Rodríguez-Calero GG, Abruña HD, Wiesner U. Block copolymer derived 3-D interpenetrating multifunctional gyroidal nanohybrids for electrical energy storage. Energy & Environmental Science. 2018;11:1261–1270. https://doi.org/10.1039/C7EE03571C. DOI: https://doi.org/10.1039/C7EE03571C Google Scholar
Chu W-C, Bastakoti BP, Kaneti YV, Li J-G, Alamri HR, Alothman ZA, Yamauchi Y, Kuo S-W. Tailored design of bicontinuous gyroid mesoporous carbon and nitrogen-doped carbon from poly(ethylene oxide-b-caprolactone) diblock copolymers. Chemistry: A European Journal. 2017;23:13734–13741. https://doi.org/10.1002/chem.201702360. DOI: https://doi.org/10.1002/chem.201702360 Google Scholar
Krüner B, Dörr TS, Shim H, Sann J, Janek J, Presser V. Gyroidal porous carbon activated with NH₃ or CO2 as lithium-sulfur battery cathodes. Batteries & Supercaps. 2018;1:83–94. https://doi.org/10.1002/batt.201800013. DOI: https://doi.org/10.1002/batt.201800013 Google Scholar
Qin Z, Jung GS, Martin-Martinez FJ, Buehler MJ. Multiscale Modeling and applications of bioinspired materials with gyroid structures. In: Shankar S, Muller R, Dunning T, Chen GH, editors. Computational Materials, Chemistry, and Biochemistry: From Bold Initiatives to the Last Mile. Cham: Springer; 2021. p. 629–644. https://doi.org/10.1007/978-3-030-18778-1_27. DOI: https://doi.org/10.1007/978-3-030-18778-1_27 Google Scholar
Garcia AE, Wang CS, Sanderson RN, McDevitt KM, Zhang Y, Valdevit L, Mumm DR, Mohraz A, Ragan R. Scalable synthesis of gyroid-inspired freestanding three-dimensional graphene architectures. Nanoscale Advances. 2019;1:3870–3882. https://doi.org/10.1039/C9NA00358D. DOI: https://doi.org/10.1039/C9NA00358D Google Scholar
Nicolaï A, Monti J, Daniels C, Meunier V. Electrolyte diffusion in gyroidal nanoporous carbon. Journal of Physical Chemistry C. 2015;119:2896–2903. https://doi.org/10.1021/jp511919d. DOI: https://doi.org/10.1021/jp511919d Google Scholar
Qin Z, Jung GS, Kang MJ, Buehler MJ. The mechanics and design of a lightweight three-dimensional graphene assembly. Science Advances. 2017;3:e1601536. https://doi.org/10.1126/sciadv.1601536. DOI: https://doi.org/10.1126/sciadv.1601536 Google Scholar
Kowalczyk P, Furmaniak S, Neimark AV, Burian A, Terzyk AP. Surface-constrained Metropolis Monte Carlo: Simulation of reactions on triply periodic minimal surfaces. Journal of Physical Chemistry A. 2024;128:1725–1735. https://doi.org/10.1021/acs.jpca.3c08203. DOI: https://doi.org/10.1021/acs.jpca.3c08203 Google Scholar
Furmaniak S. New virtual porous carbons based on carbon EDIP potential and Monte Carlo simulations. Computational Methods in Science and Technology. 2013;19:47–57. https://doi.org/10.12921/cmst.2013.19.01.47-57. DOI: https://doi.org/10.12921/cmst.2013.19.01.47-57 Google Scholar
Furmaniak S, Gauden PA, Terzyk AP, Kowalczyk P. Gyroidal nanoporous carbons – Adsorption and separation properties explored using computer simulations. Condensed Matter Physics. 2016;19:13003. https://doi.org/10.5488/CMP.19.13003. DOI: https://doi.org/10.5488/CMP.19.13003 Google Scholar
Humphrey W, Dalke A, Schulten K. VMD – Visual molecular dynamics. Journal of Molecular Graphics. 1996;14:33–38. https://doi.org/10.1016/0263-7855(96)00018-5. DOI: https://doi.org/10.1016/0263-7855(96)00018-5 Google Scholar
Bhattacharya S, Gubbins KE. Fast method for computing pore size distributions of model materials. Langmuir. 2006;22:7726–7731. https://doi.org/10.1021/la052651k. DOI: https://doi.org/10.1021/la052651k Google Scholar
P.I. Ravikovitch, A. Vishnyakov, A.V. Neimark, Density functional theory and molecular simulations of adsorption and phase transitions in nanopores, Physical Review E. 2001;64:011602. https://doi.org/10.1103/PhysRevE.64.011602. DOI: https://doi.org/10.1103/PhysRevE.64.011602 Google Scholar
Thommes M, Kaneko K, Neimark AV, Olivier JP, Rodriguez-Reinoso F, Rouquerol J, Sing KSW. Physisorption of gases, with special reference to the evaluation of surface area and pore size distribution (IUPAC Technical Report). Pure and Applied Chemistry. 2015;87:1051–1069. https://doi.org/10.1515/pac-2014-1117. DOI: https://doi.org/10.1515/pac-2014-1117 Google Scholar
Lowell S, Shields JE, Thomas MA, Thommes M, editors. Characterization of Porous Solids and Powders: Surface Area, Pore Size and Density. New York: Springer; 2004 DOI: https://doi.org/10.1007/978-1-4020-2303-3 Google Scholar
Sing K. The use of nitrogen adsorption for the characterisation of porous materials. Colloids and Surfaces A. 2001;187–188:3–9. https://doi.org/10.1016/S0927-7757(01)00612-4. DOI: https://doi.org/10.1016/S0927-7757(01)00612-4 Google Scholar
Yan Q, de Pablo JJ. Hyper-parallel tempering Monte Carlo: Application to the Lennard-Jones fluid and the restricted primitive model. Journal of Chemical Physics. 1999;111:9509–9516. https://doi.org/10.1063/1.480282. DOI: https://doi.org/10.1063/1.480282 Google Scholar
NIST Standard Reference Database Number 69. In: NIST Chemistry WebBook. National Institute of Standards and Technology, US Department of Commerce; 2023. https://doi.org/10.18434/T4D303. Google Scholar
Ribeiro MCC. Molecular dynamics simulation of liquid sulfur dioxide. Journal of Physical Chemistry B. 2006;110:8789–8797. https://doi.org/10.1021/jp060518a. DOI: https://doi.org/10.1021/jp060518a Google Scholar
Steele WA. The Interaction of Gases with Solid Surfaces. Oxfrod: Pergamon Pess; 1974. Google Scholar
Lau KF, Alper HE, Thacher TS, Stouch TR. Effects of switching functions on the behavior of liquid water in molecular dynamics simulations. Journal of Physical Chemistry. 1994;98:8785–8792. https://doi.org/10.1021/j100086a032. DOI: https://doi.org/10.1021/j100086a032 Google Scholar
Fennel CJ, Gezelter JD. Is the Ewald summation still necessary? Pairwise alternatives to the accepted standard for long-range electrostatics. Journal of Chemical Physics. 2006;124:234104. https://doi.org/10.1063/1.2206581. DOI: https://doi.org/10.1063/1.2206581 Google Scholar
Do DD, Do HD. Effects of potential models in the vapor–liquid equilibria and adsorption of simple gases on graphitized thermal carbon black. Fluid Phase Equilibria. 2005;236:169–177. https://doi.org/10.1016/j.fluid.2005.06.012. DOI: https://doi.org/10.1016/j.fluid.2005.06.012 Google Scholar
Do DD, Do HD. Modeling of adsorption on nongraphitized carbon surface: GCMC simulation studies and comparison with experimental data. Journal of Physical Chemistry B. 2006;110:17531–17538. https://doi.org/10.1021/jp062386r. DOI: https://doi.org/10.1021/jp062386r Google Scholar
Do DD. Adsorption Analysis: Equilibria and Kinetics. London: Imperial College Press; 1998. DOI: https://doi.org/10.1142/p111 Google Scholar
Jorge M, Schumacher C, Seaton NA. Simulation study of the effect of the chemical heterogeneity of activated carbon on water adsorption. Langmuir. 2002;18:9296–9306. https://doi.org/10.1021/la025846q. DOI: https://doi.org/10.1021/la025846q Google Scholar
Furmaniak S. Modelowanie adsorpcji chloroformu wewnątrz nanorurek węglowych. Cz. 1. LAB Laboratoria, Aparatura, Badania. 2014;5:15–18. Google Scholar
Kruk M, Jaroniec M, Gadkaree KP. Determination of the specific surface area and the pore size of microporous carbons from adsorption potential distributions. Langmuir. 1999;15:1442–1448. https://doi.org/10.1021/la980789f. DOI: https://doi.org/10.1021/la980789f Google Scholar
Choma J, Jaroniec M. A model-independent analysis of nitrogen adsorption isotherms on oxidized active carbons. Colloids and Surfaces A. 2001;189:103–111. https://doi.org/10.1016/S0927-7757(01)00572-6. DOI: https://doi.org/10.1016/S0927-7757(01)00572-6 Google Scholar
Choma J, Jaroniec M. Adsorption potential distributions for silicas and organosilicas. Adsorption Science & Technology. 2007;25:573–581. https://doi.org/10.1260/0263-6174.25.8.573. DOI: https://doi.org/10.1260/0263-6174.25.8.573 Google Scholar
Storn R, Price K. Differential evolution – a simple and efficient heuristic for global optimization over continuous spaces. Journal of Global Optimization. 1997;11:341–359. https://doi.org/10.1023/A:1008202821328. DOI: https://doi.org/10.1023/A:1008202821328 Google Scholar
Sips R. On the structure of a catalyst surface. Journal of Chemical Physics. 1948;16:490–495. https://doi.org/10.1063/1.1746922. DOI: https://doi.org/10.1063/1.1746922 Google Scholar
Marsh H, Rodríguez-Reinoso F. Activated Carbon. Amsterdam: Elsevier; 2006. Google Scholar
Heidarinejad Z, Dehghani MH, Heidari M, Javedan G, Ali I, Sillanpää M. Methods for preparation and activation of activated carbon: A review. Environmental Chemistry Letters. 2020;18:393–415. https://doi.org/10.1007/s10311-019-00955-0. DOI: https://doi.org/10.1007/s10311-019-00955-0 Google Scholar
Soonmin H, Kabbashi NA. Review on activated carbon: synthesis, properties and applications. International Journal of Engineering Trends and Technology. 2021;69:124–139. https://doi.org/10.14445/22315381/IJETT-V69I9P216. DOI: https://doi.org/10.14445/22315381/IJETT-V69I9P216 Google Scholar
Gao Y, Yue Q, Gao B, Li A. Insight into activated carbon from different kinds of chemical activating agents: A review. Science of the Total Environment. 2020;746:141094. https://doi.org/10.1016/j.scitotenv.2020.141094. DOI: https://doi.org/10.1016/j.scitotenv.2020.141094 Google Scholar
Sosa JA, Laines JR, García DS, Hernández R, Zappi M, de los Monteros AEE. Activated carbon: A review of residual precursors, synthesis processes, characterization techniques, and applications in the improvement of biogas. Environmental Engineering Research. 2023;28:220100. https://doi.org/10.4491/eer.2022.100. DOI: https://doi.org/10.4491/eer.2022.100 Google Scholar
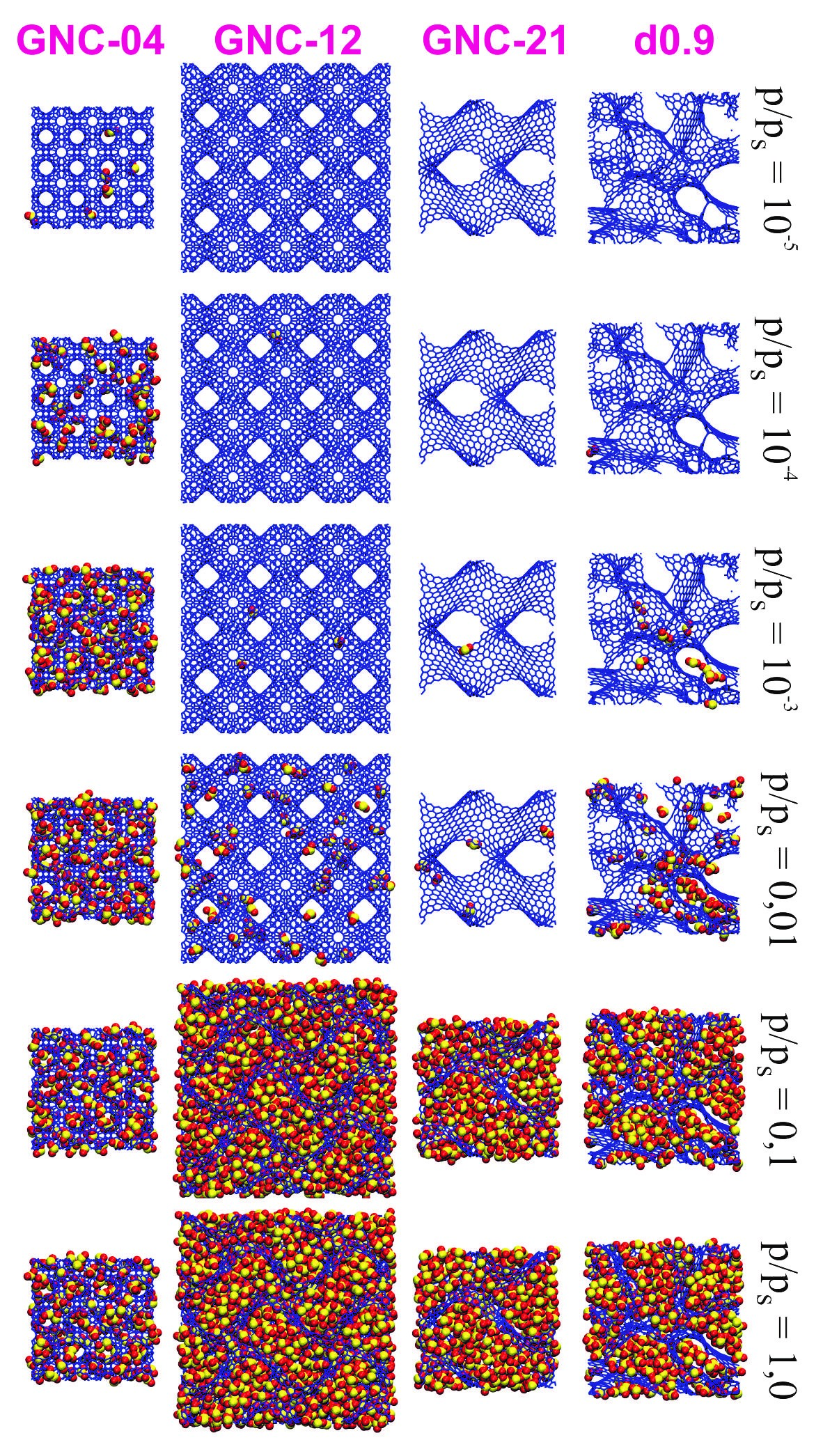
Pobrania
Opublikowane
Jak cytować
Numer
Dział
Licencja
Prawa autorskie (c) 2025 Sylwester Furmaniak, Piotr Gauden

Utwór dostępny jest na licencji Creative Commons Uznanie autorstwa – Na tych samych warunkach 4.0 Miedzynarodowe.