In silico design of self-assembly nanostructured polymer systems by multiscale molecular modeling
DOI:
https://doi.org/10.5604/01.3001.0013.4795Keywords:
multiscale molecular modeling, polymer, nanochemistryAbstract
The fast development of digitalization and computational science is opening new possibilities for a rapid design of new materials. Computational tools coupled with focused experiments can be successfully used for the design of new nanostructured materials in different sectors, particularly in the area of biomedical applications. This paper starts with a general introduction on the future of computational tools for the design of new materials and introduces the paradigm of multiscale molecular modeling. It then continues with the description of the multiscale (i.e., atomistic, mesoscale and finite element calculations) computational recipe for the prediction of novel materials and structures for biomedical applications. Finally, the comparison of in silico and experimental results on selected systems of interest in the area of life sciences is reported and discussed. The quality of the agreement obtained between virtual and real data for such complex systems indeed confirms the validity of computational tools for the design of nanostructured polymer systems for biomedical applications.
Downloads
References
Plunket JW, Plunkett's Nanotechnology & Mems Industry Almanac 2017: Nanotechnology & Mems Industry Market Research, Statistics, Trends & Leading Companies, 30 May 2017, Editor Plunkett's Nanotechnology & Mems Industry Almanac. Google Scholar
White A, The Materials Genome Initiative: One year on, MRS Bull., 2012; 37:715¬–716. Google Scholar
Hermann M, Pentek T, Otto B, Proceedings of the 49th Hawaii International Conference on System Sciences (HICSS), Koloa, HI, 2016, 3928 (doi: 10.1109/HICSS.2016.488). Google Scholar
Roco MC, Bainbridge WS, Tonn B, Whitesides G, (Eds.), Convergence of Knowledge, Technology and Society. Beyond Convergence of Nano-Bio-Info-Cognitive Technologies. Springer Verlag, 2013. Google Scholar
Scot T, Walsh A, Anderson B, O’Connor A, Economic Analysis of National Needs for Technology Infrastructure to Support the Materials Genome Initiative, 2018, Final Report, RTI International Project Number 0215231. Google Scholar
Charpentier JC, The triplet “molecular processes–product–process” engineering: the future of chemical engineering ?, Chem. Eng. Sci., 2002; 57:4667–4690. Google Scholar
Glotzer SC, Paul W, Molecular and Mesoscale Simulation Methods for Polymer Materials, Annu. Rev. Mater. Res., 2002; 32:401–436. Google Scholar
Zeng QH, Yu AB, Lu GQ, Multiscale modeling and simulation of polymer nanocomposites, Progr. Polym. Sci., 2008, 33, 191–269. Google Scholar
Jancar J, Douglas JF, Starr FW, Kumar SK, Cassgnau P, Lesser AJ, Sternstein SS, Buehler MJ, Current issues in research on structure-property relationships in polymer nanocomposites, Polymer, 2010; 51:3321–3343. Google Scholar
Yip S, Synergistic science, Nat. Mater., 2003; 2:3–5. Google Scholar
Mohanty S, Ross R, Multiscale Simulation Methods for Nanomaterials, ed. Mohanty S and Ross R, John Wiley & Sons, Hoboken, 2008, p.1. Google Scholar
Scocchi G, Posocco P, Handgraaf JW, Fraaije JGEM, Fermeglia M, Pricl S, A Complete Multiscale Modelling Approach for Polymer–Clay Nanocomposites, Chem. Eur. J., 2009; 15:7586–7592. Google Scholar
Pricl S, Posocco P, Scocchi G, Fermeglia M, Handbook of Nanophysics: Functional Nanomaterials, ed. K.D. Sattler, CRC Press, Boca Raton, 2010, pp. 3-1- 3-15. Google Scholar
Pereira SP, Scocchi G, Toth R, Posocco P, Romero-Nieto D, Pricl S, Fermeglia M, Multiscale modeling of polymer/clay nanocomposites, J. Multiscale Model., 2011; 3:151–176. Google Scholar
Toth R, Santese F, Pereira SP, Nieto DR, Pricl S, Fermeglia M, Posocco P, ize and shape matter! A multiscale molecular simulation approach to polymer nanocomposites, J. Mater. Chem., 2012; 22:5398–5409. Google Scholar
Mark F Horstemeyer, Multiscale Modeling: A Review, 2009, in Practical Aspects of Computational Chemistry, Practical Aspects of Computational Chemistry, ed. J. Leszczynski and M.K. Shukla, Springer Science+Business Media, pp. 87–135. Google Scholar
Steinhauser MO, Hiermaier S, A Review of Computational Methods in Materials Science: Examples from Shock-Wave and Polymer Physics, Int. J. Mol. Sci., 2009; 10 (12):5135–5216. Google Scholar
Curtin WA, Miller RE, Atomistic/continuum coupling in computational materials science, Modelling Simul. Mater. Sci. Eng,, 2003; 11(3):R33–R68. Google Scholar
Yeo J, Jung GS, Martín-Martínez FJ, Beem J, Qin Z, Buehler MJ, Multiscale Design of Graphyne-Based Materials for High-Performance Separation Membranes, Adv. Mater, 2019, DOI: 10.1002/adma.201805665. Google Scholar
Casalini T, Perale G, From Microscale to Macroscale: Nine Orders of Magnitude for a Comprehensive Modeling of Hydrogels for Controlled Drug Delivery, Gels, 2019; 5:28. Google Scholar
Moore G, "IEEE Technical Digest 1975" April 7, 1975, Intel Corp; "Moore's Law in perspective," 2005, Intel information sheet 306971-001US. Google Scholar
de Baas AF, What makes a material function? Let me compute the ways…, European Commission, Directorate-General for Research and Innovation, Directorate D - Industrial Technologies, Unit D3 - Advanced Materials and Nanotechnologies, Bruxelles, 2017 (doi 10.2777/417118). Google Scholar
McWeeny R, Methods of Molecular Quantum Mechanics, 2nd Edition, Academic Press, Cambridge, 1992. Google Scholar
Ercolessi F, Adams JB, Interatomic Potentials from First-Principles Calculations: The Force-Matching Method, Europhys. Lett., 1994; 26:583. Google Scholar
Fermeglia M, Ferrone M, Pricl S, Computer simulation of nylon-6/organoclay nanocomposites: prediction of the binding energy, Fluid. Phase. Equilib., 2003; 212:315–329. Google Scholar
Vv. Aa., Molecular Simulations and Industrial Applications, ed. K.E. Gubbins and N. Quirke, Gordon & Breach, Amsterdam, 1996. Google Scholar
Chen JC, Kim AS, Brownian Dynamics, Molecular Dynamics, and Monte Carlo modeling of colloidal systems, Adv. Colloid Interface Sci., 2004; 112:159–173. Google Scholar
Hoogerbrugge PJ, Koelman JMVA, Simulating Microscopic Hydrodynamic Phenomena with Dissipative Particle Dynamics, Europhys. Lett., 1992; 19:155. Google Scholar
Groot RD, Warren PB, Dissipative particle dynamics: Bridging the gap between atomistic and mesoscopic simulation, J. Chem. Phys. 1997; 107:4423. Google Scholar
Chen S, Doolen GD, Lattice Boltzmann Method for Fluid Flows, Annu. Rev. Fluid Mech., 1998; 30:329-364. Google Scholar
Glotzer SC, in Annual Reviews of Computational Physics, ed. Stauffer D, World Scientific, Singapore, pp. 1–46. Google Scholar
Fraaije JGEM, van Vlimmeren BAC, Maurits NM, Postma M, Evers OA, Hoffmann C, Altevogt P, Goldbeck-Wood G, The dynamic mean-field density functional method and its application to the mesoscopic dynamics of quenched block copolymer melts, J. Chem. Phys. 1997; 106:4260. Google Scholar
Hughes TJR, The Finite Element Method, Prentice-Hall, Englewood Cliffs, 1987. Google Scholar
Gusev AA, Numerical Identification of the Potential of Whisker- and Platelet-Filled Polymers, Macromolecules, 2001; 34:3081–3093. Google Scholar
Goddard III WA, Cagin T, Blanco M, Vaidehi N, Dasgupta S, Floriano W, Belmares M, Kua J, Zamanakos G, Kashihara S, Iotov M, Gao G, Strategies for multiscale and simulation of organic materials: polymers and biopolymers, Comput. Theor. Polym. Sci., 2011; 11:329–343. Google Scholar
McGrother S, Goldbeck-Wood G, Lam YM, Integration of modelling at various length and time scales, Lect. Notes Phys., 2004; 642:223–230. Google Scholar
Scocchi G, Posocco P, Fermeglia M, Pricl S, Polymer−Clay Nanocomposites: A Multiscale Molecular Modeling Approach, J. Phys. Chem. B, 2007, 111, 2143–2151. Google Scholar
Cosoli P, Scocchi G, Pricl S, Fermeglia M, Many-scale molecular simulation for ABS–MMT nanocomposites: Upgrading of industrial scraps, Microporous Mesoporous Mater., 2008; 107:169–179. Google Scholar
Fermeglia M, Pricl S, Multiscale molecular modeling in nanostructured material design and process system engineering, Comput. Chem. Eng., 2009; 33:1701–1710. Google Scholar
Doi M, Material modeling platform, J. Comput. Appl. Math., 2002; 149:13–25. Google Scholar
Milano G, Müller-Plathe F, Mapping Atomistic Simulations to Mesoscopic Models: A Systematic Coarse-Graining Procedure for Vinyl Polymer Chains, J. Phys. Chem. B, 2005; 109:18609–18619. Google Scholar
Toth R, Voorn DJ, Handgraaf JW, Fraaije JGEM, Fermeglia M, Pricl S, Posocco P, Multiscale Computer Simulation Studies of Water-Based Montmorillonite/Poly(ethylene oxide) Nanocomposites, Macromolecules, 2009; 42:8260–8270. Google Scholar
Ghanbari A, Ndoro TVM, Leroy F, Rahimi M, Böhm MC, Müller-Plathe F, Macromolecules, Interphase Structure in Silica–Polystyrene Nanocomposites: A Coarse-Grained Molecular Dynamics Study, 2012; 45:572–584. Google Scholar
Müller-Plathe F, Coarse‐Graining in Polymer Simulation: From the Atomistic to the Mesoscopic Scale and Back, Chem. Phys. Chem., 2002; 3:754–769. Google Scholar
Fermeglia M, Pricl S, Multiscale modeling for polymer systems of industrial interest, Prog. Org. Coat., 2007; 5:187–199. Google Scholar
Posocco P, Fermeglia M, Pricl S, Morphology prediction of block copolymers for drug delivery by mesoscale simulations, J. Mat. Chem., 2010; 20:7742–7753. Google Scholar
Posocco P, Gentilini C, Bidoggia S, Pace A, Franchi P, Lucarini M, Fermeglia M, Pricl S, Pasquato L, ACS Nano, 2012; 6:7243–7253. Google Scholar
Pengo P, Sologan M, Pasquato L, Guida F, Pacor S, Tossi A, Stellacci F, Marson D, Boccardo S, Pricl S, Posocco P, Gold nanoparticles with patterned surface monolayers for nanomedicine: current perspectives, Eur. Biophys. J., 2017; 46:749–77. Google Scholar
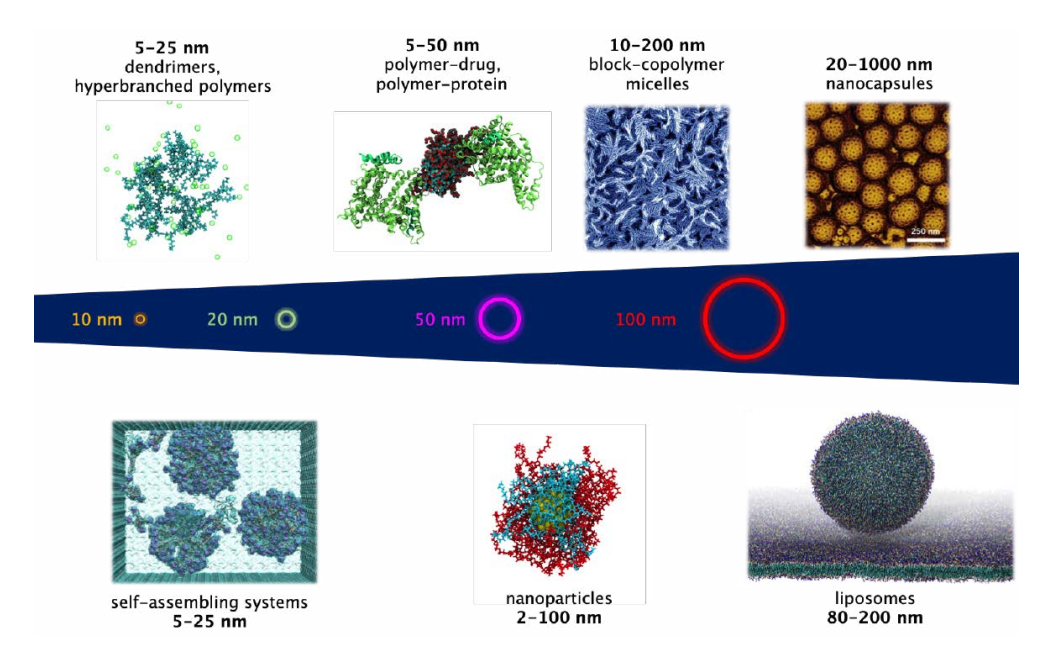
Downloads
Published
How to Cite
Issue
Section
License
Copyright (c) 2019 University of Applied Sciences in Tarnow, Poland & Authors

This work is licensed under a Creative Commons Attribution-NonCommercial 4.0 International License.